An Assessment of Flow-Based Market Coupling in the Nordics

Our Market Expert for the region, Priyanka Shinde takes an in-depth look at the advantages and drawbacks of Flow-Based Market Coupling ahead of its October 2024 implementation.
Over the past few years, several EU directives have begun laying the foundations to allow the European electricity market to operate in an intercoupled manner. The European electricity grid is intercoupled, with several countries operating as a part of synchronous areas.
As the trades that take place in the electricity market are connected to the real-time flow of electricity, it is essential to account for the physics of the system while trading in the markets - due to its relation to the system frequency and thereby the stability of the underlying power system.
This article will explain how the electricity grid and market coordinate with each other in the Nordic market. Using observations from how the cross-border integration of electricity markets has evolved in the central Europe, we will also explore the changes we expect to see in the Nordic region. With variable renewable energy sources accounting for a larger part of the energy mix, the challenges this poses to the coordination of grids and markets is also a major topic of focus.
The start of flow-based coupling
The first flow-based market coupling (FBMC) project in Europe was introduces in May 2015, when the Central Western Europe (CWE) capacity calculation region adopted this method. It included Belgian, Dutch, French, German/Luxembourgian and (since 2018) Austrian bidding zones. The CWE FBMC ran on a daily basis, gathering inputs from its participating Transmission System Operators (TSOs) and Nominated Electricity Market Operators (NEMOs). Until 7 June 2022, prices and net positions were calculated for these five bidding zones, aiming to minimise costs while respecting operational security.
The Core Day-Ahead Flow-Based Market Coupling Project was successfully launched on 8th June 2022, integrating the electricity grids and markets of 12 bidding zones across 13 European Member States. The main objective of this project has always been to enhance cross-zonal exchanges, improve grid security, increase price convergence, and strengthen market resilience to accommodate large-scale renewable energy integration.
This initiative brought together 16 TSOs and 10 NEMOs to create a unified market coupling process. As a flagship project for short-term market integration in Europe, it supports the objectives of the Third Energy Package and the Clean Energy Package.
However, in the Nordics, discussions around implementing a flow-based method are about 10 years old. The planned go-live for Nordic flow-based market coupling is October 2024. Below, I have written about how the calculations work in order to provide some much needed context an information. However, if you would like to skip to the part about the implementation of FBMC in Nordics (as well as my analysis of the parallel run results published by the Nordic Regional Coordination Centre (RCC) for the past 18 months) please scroll down to the section titled: Nordic RCCs parallel runs analysis.
Transmission capacity allocation methods
Before moving into the technical details, it would be useful to outline some key terms.
Available Transfer Capacity (ATC)
In an ATC world, the link between commercial transactions and the physical characteristics of the electricity grid is simplified. In order to calculate the ATC, the TSOs consider forecasts available two days before the delivery of electricity. They then guess the market outcomes and allocate capacities based on their experience. The capacity allocation in this method takes place before the market clearing. The ATC is calculated as the maximum commercial exchange between two market areas, compatible with the physical transmission constraint and operational security standards. The ATC of a cross-border link is independent of the flow on other cross-border links. The drawback of this method is that it limits the full exploitation of the underlying available transmission capacities.
Flow-based Market Coupling (FBMC)
Going back to the basics of electrical engineering, we can say that power injection reaches the source by passing through the network based on the impedance offered. Fig. 1 shows an example where 1000 MW of injection at node A is split over lines A to B to C and A to C based on the characteristics of these lines. In this case, we call these lines Critical Network Element with Contingency (CNEC). A Critical Network Element (CNE) is a line that limits the amount of electricity that can be transmitted across the network. These elements are critical because they are the bottlenecks or constraints that could limit the available capacity for cross-border electricity exchange. Critical Network Element with Contingency (CNEC) is a CNE considered together with a specific contingency scenario.
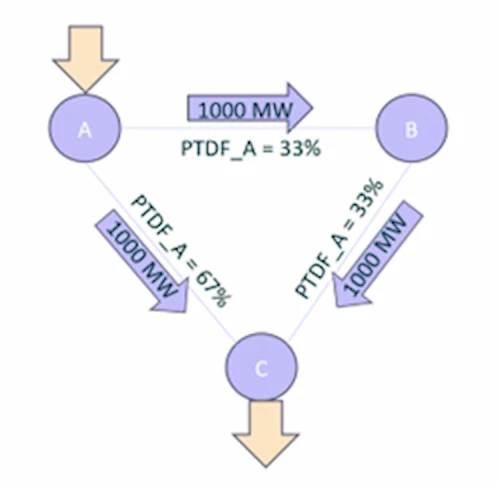
Imagine the same network as in Fig.1, but with the injection of 1000 MW at node A, the follow in element A to C changed by 670 MW. This would mean that the sensitivity of element A to C to a change in the net position of node A is 0.67. This sensitivity is called the Power Transfer Distribution Factor (PTDF). It is defined for each line with respect to every node.
In order to apply this to the real-world, think about node A as a bidding zone (with many nodes within the zone). We then need know how will an individual node affect the overall bidding zone position. This can be calculated using a Generation Shift Key (GSK) factor which can vary based on the strategy followed.
Flow-based market coupling is a two step process: Capacity calculation and capacity allocation as shown in Fig. 2.
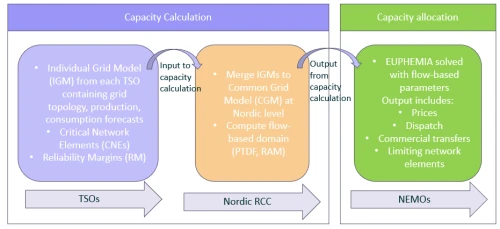
Capacity calculation - how to do it
TSO selection of Critical Network Elements
Transmission System Operators (TSOs) identify network elements they consider critical for capacity calculations. For these elements, TSOs establish maximum flow limits based on operational security thresholds.
Coordinated Capacity Calculation
Nordic RCCs employ the flow-based method to assess how changes in consumption or production within a bidding zone impact the flow on these critical network elements. This relationship is assumed to be linear, meaning the flow changes proportionally to variations in consumption or production.
TSO validation and market optimisation
TSOs validate the flow-based parameters, and the results are provided to market operators. The market operators then optimise net positions for all bidding zones, ensuring that none of the critical network elements exceed their capacity limits due to pan-European Day-Ahead market optimisation.
Capacity allocation - how it works
As a capacity allocation mechanism, the Flow-Based (FB) method maximises the welfare of exchanges within and between bidding zones, considering the constraints imposed by critical network elements under normal (n state) and outage conditions (n-1 state), where one transmission element is unavailable on the allocation day.
In FB, the entire domain is considered as a whole. This mans that increasing flows by 1 MW in one bidding zone creates a knock-on impact for net positions across all zones, even those not directly connected. By contrast, ATC calculates net positions based on bilateral constraints, maximising exchanges per border.
FBMC optimisation output
The output of the FBMC optimization is a set of zonal net positions and corresponding market clearing prices. The optimisation seeks the combination of net positions that maximises social welfare.
FB domain and constraints
The FB domain is defined by a set of constraints. Each constraint represents a critical line where flow could be affected by changes in net positions via the PTDF matrix. Physical flows on each line must stay within the commercially available capacity, known as RAMs.
EUPHEMIA's role
These flow-based parameters are then given as inputs to EUPHEMIA, the market coupling algorithm. which uses a heuristic approach to find the best solution within 17 minutes (will be extended to 30 minutes to support 15 minutes Market Time Units (MTUs)), although the non-linear nature of the problem makes it challenging to achieve a fully optimal solution. This results in the net positions of each bidding zone based on the allocation from EUPHEMIA. The outcome also includes prices, dispatch, commercial transfers between bidding zones and limiting elements in the network.
Visualising the margins
In simple terms, a flow-based market coupling mechanism calculates available capacity for cross-zonal exchanges to maximise social welfare. Generally, increasing cross-zonal exchanges boosts welfare, but this is also limited by the available capacity on the transmission network. This capacity is calculated based on the following factors: Fmax (maximum power that can be transferred considering thermal capacity), FRM (Flow Reliability Margin to manage deviations due to forecast uncertainties), F0 (flow on the CNE in case all bidding zones are set to zero net position or in other words linearization from base case at zero net position (internal flow)), RA (Impact of remedial actions on flow on the CNE), AMR (Adjustment for minimum RAM is used to ensure that RAM is always greater than or equal to 0), AAC (Already allocated capacity on the CNE is used to represent allocations for frequency restorative reserves), and IVA (Individual validation adjustment provided by TSOs during domain validation in case of unplanned outages or incorrect input data is detected). The remaining available margin (RAM) is calculated as:
RAM = Fmax – FRM – F0 – AAC + RA + AMR - IVA
A ternary plot helps to visualise this by showing the relationship between three elements, RAM, F0, and FRM’ (= FRM – RA – AMR + AAC + IVA), as a triangle. The next figure uses this method to display data from the Nordic FBMC parallel runs. The figures show the average values for the respective months they are plotted for - as indicated in their titles. These ternary plots are inspired by the amazing work of Nico Schoutteet in the Central Western Europe and Core regions.
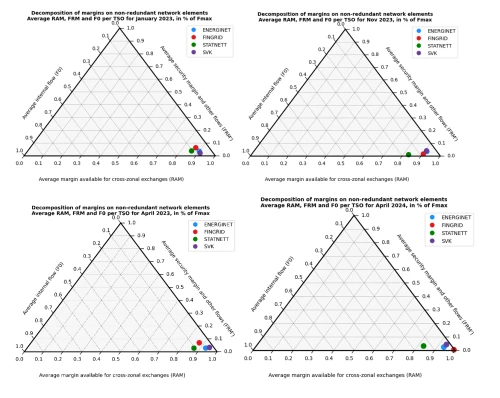
Bidding zones in the Nordics are more established, with borders placed at historically congested grid points. They also have more (smaller) bidding zones. This results in higher RAMs for all the months plotted in Fig. 3.
RAM can occasionally exceed 100% when the F0 is negative. It would mean the trades would first have to negate that F0 flow and then can add up to the RAM. On averaging these values of F0 and RAM over a month, we can obtain results as seen for Fingrid in April 2024. (Note that I have not removed any nonredundant CNECs while plotting these ternary plots. All the CNECs belonging to any of the four TSOs are considered while determining the average values shown.)
More on the flow-based domains
Based on the PTDF data available from JAO, we obtain the zone-to-slack PTDF which gives the sensitivity of a CNEC to the change in net position of a bidding zone. In order to obtain zone-to-zone PTDF, we subtract the zone-to-slack PTDFs.
At this point, it is important to highlight that while there are 12 real bidding zones in the Nordics, the HVDC links are also modelled as bidding zones in the flow-based methodology as shown in Fig. 4. There are therefore 19 virtual bidding zones, thereby making the entire bidding zone count for FBMC to be 31. Note that the North Sea Link and Viking cable are excluded from the virtual bidding zones as they do not appear into the SDAC.
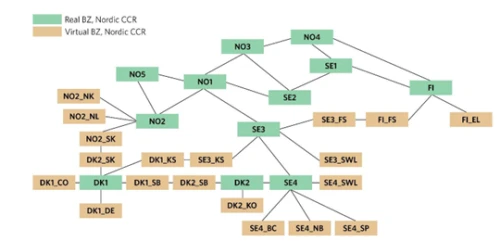
Flow-based domain using net positions:
The first method to visualize the flow-based domain relies on the number of bidding zones, in this case 31. Therefore, we would have a 31-dimensional domain. It means that the net position of each bidding zone can lie within this polyhedral given the combined margins of all pre-solved CNECs.
It would be too ambitious to visualize this domain, so we start with a two-dimensional space by taking the cross-section of the larger domain. For example, for a combination of SE3 and NO5, we obtain the presolved CNECs for 14th Sept and 14th Nov 2023 from 20:00 to 21:00 CET. The resulting plots can be obtained by PTDFs of both these areas corresponding to the nonredundant CNECs and RAMs. The resulting domains and net positions are shown in Fig. 5.
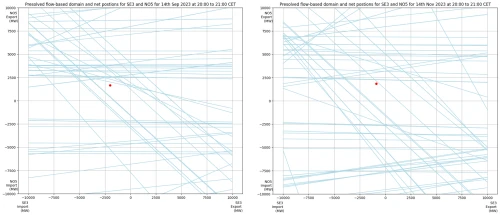
As we select these two bidding zones, we are able to view one of the 930 (n*(n-1)) combinations possible for this hour. The domains of two different dates are visualised, so they do vary from each other while the net position in each of these cases are also different.
Flow-based domain using bilateral exchanges:
These zone-to-zone PTDFs are useful in creating the flow-based domain for set of borders which gives the bilateral scheduled exchanges. The total number of cross-border lines would be 37 (as seen in Fig. 4). Therefore, it results in a 37-dimensional space for visualising the flow-based method for exchanges. We consider the flows in between SE3 to SE2 and SE3 to SE4 in this example to limit it to two-dimensional space. For these domains, we consider the zone-to-zone PTDF to calculate the slopes.
The schedules exchanges between SE3-SE2 and SE3-SE4 are indicated with the red dots in both the plots in Fig. 6.

Nordic RCCs parallel runs analysis
While it is interesting to observe the complexity of the flow-based capacity calculation method, it is equally intriguing to find out the effect of such an allocation on the prices, net positions and flows in between different bidding zones.
Therefore, in this section, I have analysed the data published by the Nordic RCCs parallel runs (an experiment to see how things would have been different under the FBMC method) over the past 18 months.
In Fig. 7, we compare the percentage flows through ATC and FBMC in between certain Nordic bidding zones. For the ease of comparison and plotting, the flows are converted into percentages by dividing the flows to the maximum average flow observed on the same line in either of the two cases across the dataset.

The seasonal patterns are captured in the Fig. 7 for the flows where FBMC seems to provide larger percentage of flows compared to ATC in most months. In the winter months, the flows are higher on these lines and FBMC allows for larger percentage of flows. It is interesting to observe that the flow direction changes from the SE3-FI bidding zones as the method is changed.
In April/May 2023, these flows might have been driven by Olkiluoto 3 coming online. Again, this pattern was observed in June 2024 when FI to SE1 flows were observed in the FBMC method. SE3 to NO1 flows were also reversed in ATC compared to FBMC for June to October in 2023. In FBMC, the flow direction for SE3 to NO1 remained consistent throughout the period with overall percentages showing higher for each month - which explains some of the results we see below.
Fig. 8 shows the average of the net positions from ATC and FBMC for the data published from 12 December 22 until 30 June 2024. The positive values in net position indicate that the zone is net exporting while negative value indicates imports.
We can observe more changes in the net positions in certain areas - for example in Norway. As FBMC releases more capacities on certain bidding zones, it allows for reallocation of resources such that more flows are possible from cheaper zones to expensive ones.
The impact on NO2 can be partly explained by the increase of flow from NO1 to NO2 made possible by FBMC. The flow-based simulation results are based on NTC bids (the bids will change when flow-based goes live). The producers in NO2 would also need to adjust their water value to reflect the new capacities provided by flow-based.
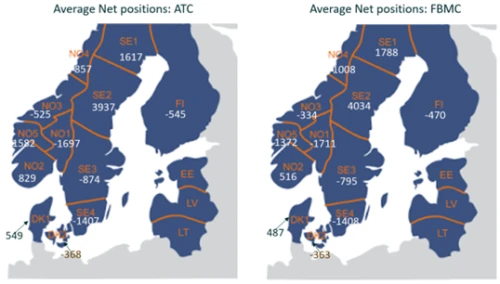
Table 1 shows the average price differences for different bidding zones combinations. This shows that price differences reduce in FBMC compared to ATC on most bidding zone border combinations. This indicates that FBMC is a more efficient in allocating capacities, compared to the ATC method, leading to more price convergence - one of the EU directive's stated aims.

We can also look into the spreads in each of the 12 bidding zones (the real ones) to understand how the change in capacity calculation method influences the volatility in a given bidding zone.
Fig. 9 shows the average monthly spreads calculated with the ATC and FBMC prices per bidding zone. I have excluded the data from December 2022 as it was only partially available and more volatile. The spreads are calculated by taking the maximum and minimum of the prices for each day averaged out per month for each bidding zone. We can see that FBMC reduces the spreads in most areas and seasons. This gives an indication of lower market volatility as a result of changes in the capacity calculation methodology. As there are more cross-border flows possible in FBMC, it might also reduce the need for expensive generation to be used to balance the system when it is short.

Building on the market volatility analysis, we can also study hourly price patterns and look into negative price situations in Fig. 10. The average prices from both the capacity calculation methods are compared in the plots.
The prices in NO3 during the winter months have higher peaks (and higher prices in general) for most hours with ATC compared to FBMC. The trend is similar in the summer months, while for negative price hours, the negative peaks are more extreme under the ATC methodology compared to FBMC. This is due to FBMC facilitating further flows and releasing more capacities.
For SE3, in the winter months, while most hours have similar maximum prices for both the methods, the morning and evening peak hours observe higher maximum prices in FBMC compared to ATC method. In the summer months, on the other hand, the maximum prices are lower in FBMC compared to ATC. Negative price hours are also less frequent with FBMC in SE3.

The principle of the flow-based method is to allocate resources in such a way that it improves the social welfare. The capacities are allocated to facilitate the flow from price zones with cheaper resources to the ones with expensive resources. It is important to compare how this influences the relation of the prices in different bidding zones.
Comparing the two heatmaps in Fig. 11, we observe that the correlation between the prices does increase in most cases, but not all with FBMC. For example, in NO3, the correlation with SE1, SE2 and NO4 reduces, while it improves with SE4, NO1, NO2, NO5.
NO1 and NO5 seem to get higher correlation with SE3 and SE4 under FBMC. DK1 and DK2 also have more correlation with the Norwegian zones with FBMC compared to ATC. It indicates that the improved capacities towards areas with more demand in the southern parts lead to more flows in that direction and higher price correlation through the FBMC method compared to ATC.

Qualitative Analysis of FBMC
When it comes to the potential advantages and disadvantages of changing to FBMC, market participants and Nordic TSOs have been discussing a range of topics:
Price differences:
As the flow-based methodology hopes to achieve overall higher social welfare in the market, it promotes efficient use of resources, strengthens the market coupling, and leads to more price convergence. However, it also means higher prices in some price zones and lower in others.
Counterintuitive flows:
Counterintuitive flows are the ones which flow from higher price zones to lower price zones. In the flow-based method, changing net positions in certain bidding zones to bring higher social welfare across the market could lead to some cases where such counterintuitive flows arise.
Hydro water values:
In a system with large amount of hydropower, the prices are affected by water values. As the capacities between bidding zones are changed in the flow-based method, water values are also affected. Updated water values, will in turn affect bidding strategies, resulting in a change in prices. It is important to note therefore, that the simulated prices from the parallel runs do not entirely reflect the reality.
Reduced intraday capacities:
As far as the Nordic flow-based market coupling project is concerned, there have been concerns on the intraday market that capacities that will be left over after the flow-based method is applied for the Day-Ahead capacity calculation.
In a recent stakeholder meeting, Nordic TSOs presented a few extreme market events where they illustrated that capacities would have been sufficient in the case of a flow-based methodology being implemented. This method of ATCE is an interim method. The aim is to eventually develop this further to do a recalculation of flow-based domains after the day-ahead market as applied in the CORE region in May 2024 with IDCC(b). This recalculation will be based on updated grid model forecasts.
Moreover, the trading pattern could also shift if the traders observe that often they do not have sufficient volumes to trade their imbalances in intraday markets, meaning that they could adjust their behaviour across the trading timeline. There will also be learnings on how the net positions changes in certain bidding zones affect the CNEC flows and thereby the dispatch decisions.
I am planning to continue my analysis of ID ATCE and present it at our Short-term Power Market Seminar, so stay tuned!
Impact on Balancing markets:
In the Nordics, we have a single imbalance pricing system, designed in a way to help balance the system better. Therefore, with updated capacities and opportunities to balance out the system, it will be important to study the impact on resulting imbalance prices and overall system balancing costs. In the last stakeholder meeting in June 2024, this topic was raised and we could expect some more understanding on this matter in the coming months
Conclusion:
With the aim to enhance cross-zonal exchanges, improve grid security, increase price convergence, and strengthen market resilience to accommodate large-scale renewable energy integration, flow-based market coupling is set to go-live in the Nordics in October 2024.
In this article, I have looked into the market outcomes, price spreads, net positions, resulting flows and summarized the findings from the Nordic TSOs parallel runs taking place before go-live. The results for the day-ahead market do indicate that flow-based market coupling facilitates higher exchanges, in a way that reduce the price spreads across Nordics and reduces volatility compared to the ATC method used today.
However, as the same bids as in the ATC world are utilised for the parallel runs, the bidding behaviour changes we would expect to see as a result of changes to the capacity methodology is ignored. In a hydro-dominated system like Nordics, the changes in water values due to the new capacities will also play a role in setting the prices. The impact on intraday and balancing markets is also important to observe.
As FBMC has been operational in the central Europe for several years now, it has led to detailed research work and subsequent improvements with time. Similarly, in the Nordics, we have just started to see some research work being pursued recently. However, once the project is implemented and the real data is available, it will lead to further research and improvements will follow.
Follow market changes as they happen